Mutants and mutations - The Uncanny X-Flies
In this post we will talk about mutants. What is a mutant? What is a mutation? How do mutations occur? What are their consequences? How can we use them? What is their importance in nature? (it is also an excuse for me to mix in my favourite pop-culture mutants and pay homage to their creators Stan Lee and Jack Kirby).
In many of the past articles we talked about the importance of analyzing mutants in research done with Drosophila flies. We have mentioned that TH Morgan discovered one white-eyed fly amongst thousands of normal red-eyed flies, leading him and his team to later establish that the hereditary information -the genes- is in the chromosomes -molecules of DNA.
It was HJ Muller, one of Morgan’s former collaborators who demonstrated in 1929 that X-Rays provoked mutations, and could modify the structure of the chromosomes and provoke rearrangements of pieces of the chromosomes.
Let’s dive in a little deeper.
What is a mutation? What is a mutant?
A mutation is a change in the genetic characteristics that has been passed down from a progenitor to its descendent. A mutant is the cell or organism that carries those changes in its genome. This means that a mutant has different genetic characteristics than its parents and could potentially inherit them to its progeny.
A mutant can be a cell or a whole organism. As we have mentioned when we talked about transgenesis techniques, pluricellular organisms that reproduce sexually -such as us and flies- have two big categories of cells; somatic cells, those that make up the body (soma means body in greek) and germ cells, which are the special cells that will form the eggs and sperm to produce new organisms when they are combined.
A mutation in a somatic cell will be passed down only to its daughter cells when it divides and to the further descendants of those daughter cells in subsequent generations of cells. They will form a population of mutant cells in the body (more on this later).
A mutation in the germ line on the other hand, can be passed to the next generations of organisms. The fly that comes from a mutant egg or a mutant sperm will carry the mutation in all of its cells, because all of those cells are descendants of that single mutant egg or sperm.
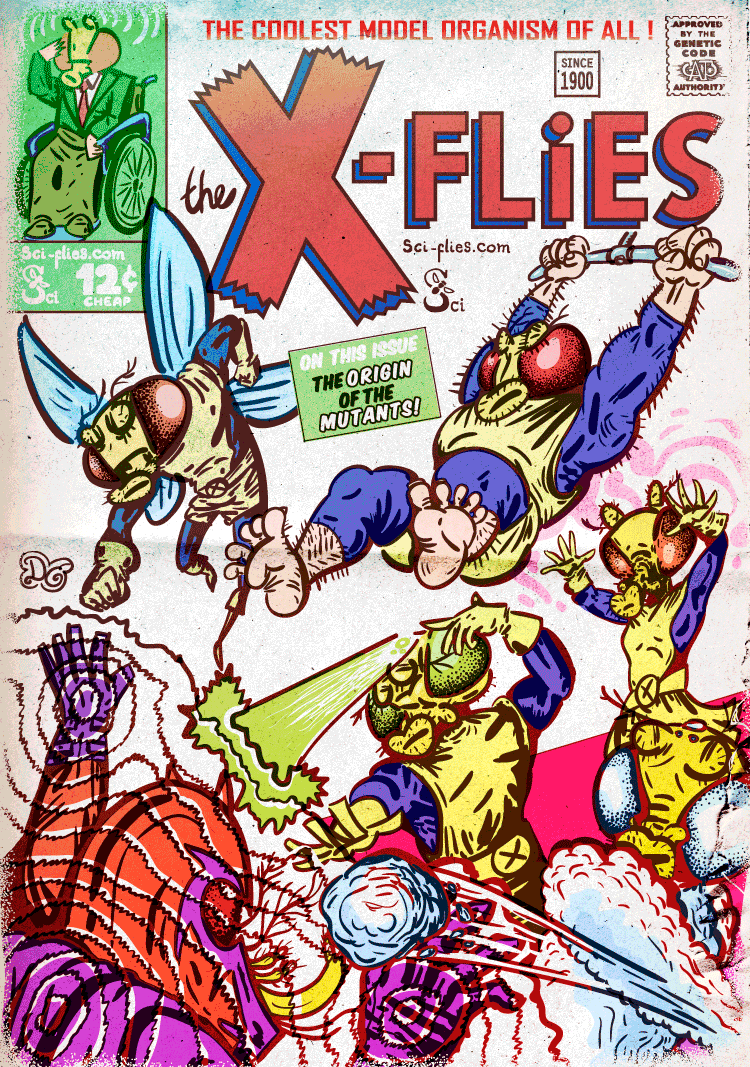
Types of mutations - how they occur
So, mutations are alterations on the sequence of the DNA. These changes can be of many different types, they can be caused by different means and have different consequences.
What types of changes can occur?
As we mentioned when we talked conceptually about DNA and how it works, the DNA molecule is made of two long non-repetitive sequences of units (called bases) that come in four different types A, T, C and G (in that post I had used colors to abstract them). The two single strands of bases that form a molecule of DNA are kept together because the bases join each other in pairs; A with T and C with G. The two strands have complementary sequences, that is to say that where one has an A the other has a T, where one has a C the other a G, and vice versa, so they match each other.
Mutations may be as simple as single base substitutions, in which a base in the original sequence has been changed for either of the other three. They can also be insertions or deletions, in which the mutant either has new bases added or some bases removed from the sequence. There can also be rearrangements of pieces of the sequence.
How do they occur?
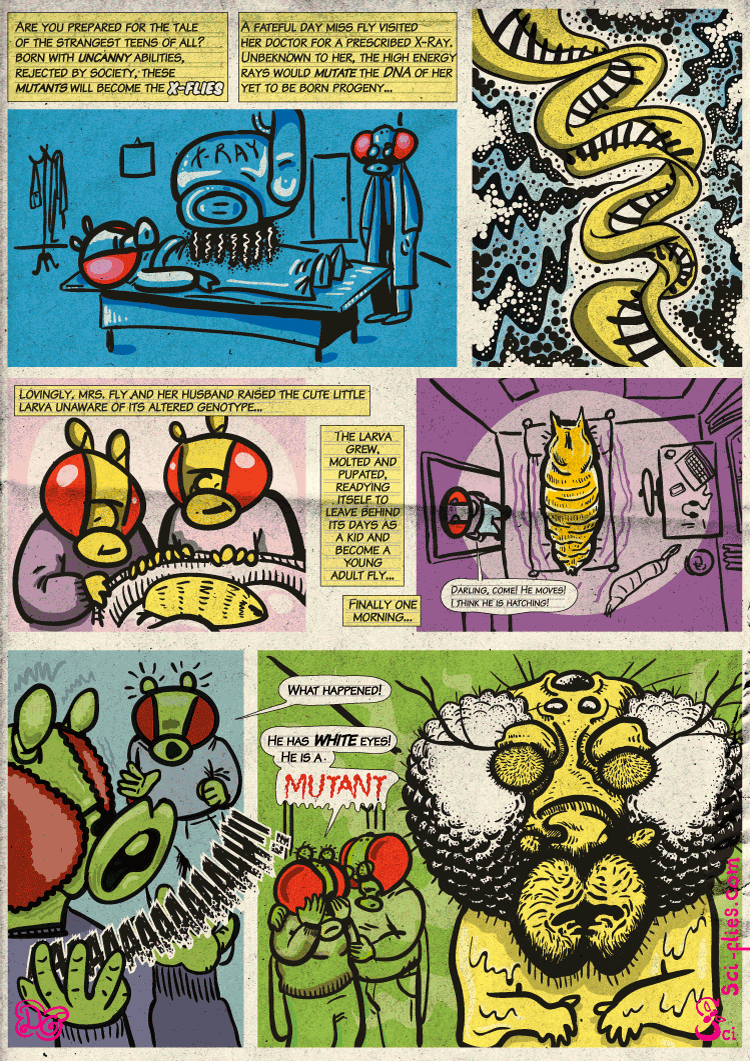
Spontaneous mutations
Mutations can happen spontaneously through the normal functioning of DNA. During the replication process DNA is unraveled and each single strand is used as the mold to build a new strand, by sequentially adding the pairing bases one by one.
Spontaneous mutations may involve this process of DNA replication. Although the replication process is very efficient, errors do happen. Bases may be wrongly paired and an incorrect one introduced. It can occur that the mold strand slips and a base that was already added is added again or it can provoke that a base is wrongly skipped. Spontaneous mutations can also occur due to previous chemical changes in the bases on the DNA strand, that later results in a wrong reading during replication and the building of a wrong new strand.
Mutagens - Chemicals
As we mentioned already, mutations can be induced by environmental factors such as chemicals and radiation. Factors that can alter DNA sequence and produce mutations are called mutagens. How do mutagens alter DNA?
There are many chemical mutagens that act in different ways. Some are chemically similar to the bases (they are called base analogs) and can be incorporated into DNA during replication. Since they are similar but not the same, in subsequent replications the may provoke the pairing with wrong bases in its place.
Other chemicals provoke chemical modifications to the bases already in the DNA, which induce errors also by wrong pairing later on during replication. For example, EMS is a widely used chemical in Drosophila research to generate random mutations (it is the one that Konopka and Benzer had used, for example).
Some mutagenic chemicals are only found in the environment either naturally or artificially made by humans. There are also some mutagenic molecules that occur naturally in the organism as products of its normal functioning, this is the case of some molecules called oxidative agents (reactive oxygen species) which are produced by the cell during the normal use of oxygen to produce energy (of course the cells have mechanisms to clear these molecules and protect from their effects).
Other chemicals provoke mutations by getting in between the bases (intercalating) which alters the shape of DNA and provokes jumps and skips during replication instead of wrong pairings.
Mustard gas, which was used as a weapon during the World War I, was the first chemical demonstrated to be a mutagen. This discovery was made by Charlotte Auerbach working on Drosophila flies.
Charlotte was Jewish born in Germany in 1899. There she studied Biology, Chemistry and Physics. She started as a secondary school teacher but antisemitism in the classroom made her quit. She decided to do a PhD but she did not like the project or the treatment she received, so she went back to teaching children. That did not last long because in 1933 all Jewish teachers were dismissed. She left Germany for Edinburgh.
In Edinburgh she was able to complete her PhD. There she studied the development of the fly’s legs. After that she worked as a lab assistant. It was not a good job but allowed her contact with very influential scientists. In 1938 she met Hermann Muller. She wanted to work on understanding how genes are involved in development. He taught her that in order to understand how genes work it is necessary to see what happens when they are mutated.
Muller had worked on the effects of X-Ray radiation on genes. He had seen a report on the similarities of the lesions caused by mustard gas to those of X-Rays and suggested that maybe chemicals could also act as mutagens. This led to Charlotte working with JM Robson on the effects of mustard gas on the genetic material of Drosophila flies and the first demonstration of chemically induced mutations.
She could not publish her results until 1946 because the British government had feared they might have been used against them during the Second World War.
Charlotte continued studying the mutagenic effects of chemicals for many more years.
Mutagens - Radiation
Radiation is energy travelling through space. For example, light is a form of radiation (called electromagnetic radiation). White light, like the one we can see and receive from the sun, is composed of rays of different intensities of energy. A way of observing these different rays is by separating them. We see this every time it rains during sunshine and rainbows form. The different colors of the rainbow are the rays of different energy. Towards the red end, they are less energetic while the other end, the violet, is more energetic.
When radiations below the violet hit us, we feel heat, and nothing more. But radiations beyond the violet have higher energies that are enough to allow reactions to happen on molecules and atoms. They are called ionizing radiations for this reason.
Immediately beyond violet is Ultraviolet (UV) radiation. The sun bathes us with UV radiation, but we cannot see it. UV radiation can act directly as a mutagen. It has enough energy to provoke chemical reactions within DNA. It can provoke that two T (or C) bases that are next to each other on the same strand to join, generating a bulge in the DNA molecule that can lead to faulty replication.
Beyond UV are radiations with higher energies. These are X-Rays and Gamma Rays (I should have drawn the Hulk, maybe?). The energy of these radiations allows them to penetrate and go through biological tissues (that is why X-Rays can be used to see through our bodies). The energy of these radiations provokes molecules to turn unstable and in the case of DNA can cause many of the same effects already mentioned and even break bonds between molecules and provoke breaks in the DNA molecule.
Beyond the energy levels of X-Rays and Gamma Rays radioactive materials emit alpha, beta and neutron particles which have even higher energy and different penetration power.
It is important to note that DNA damage and mutagenesis are not the same. DNA damage can be so strong that the cell cannot stand it and dies. But DNA damage can be a source of mutation if the repair process introduces errors.
Radiations can be mutagenic in an indirect way, by generating reactive molecules (such as the reactive oxygen species) that in turn modify DNA.
Finally, another source of mutation are transposable elements. We have talked about these pieces of DNA that can change place in the genome. When they move, they can leave DNA behind where they used to be. They can also carry with them pieces of DNA that were around them. Also, they can also provoke changes in the place where they land. In their mobilization they may interrupt gene or regulatory sequences. More than half of spontaneous mutations in Drosophila occur through transposable elements that jump around and land in or near genes and regulatory regions.
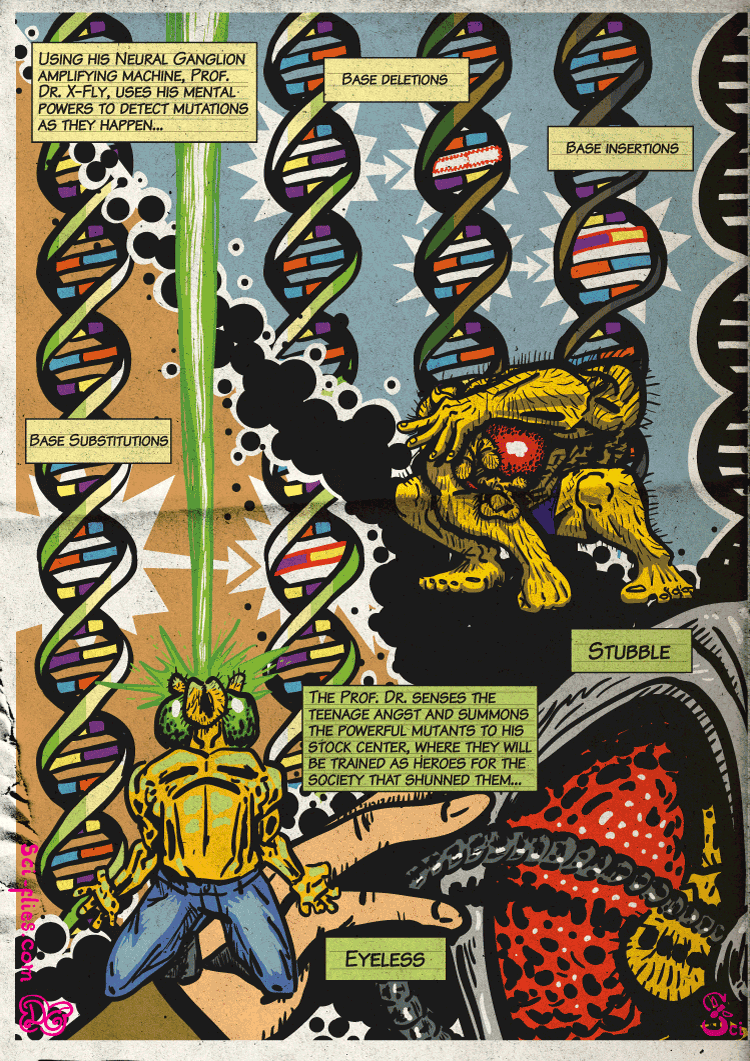
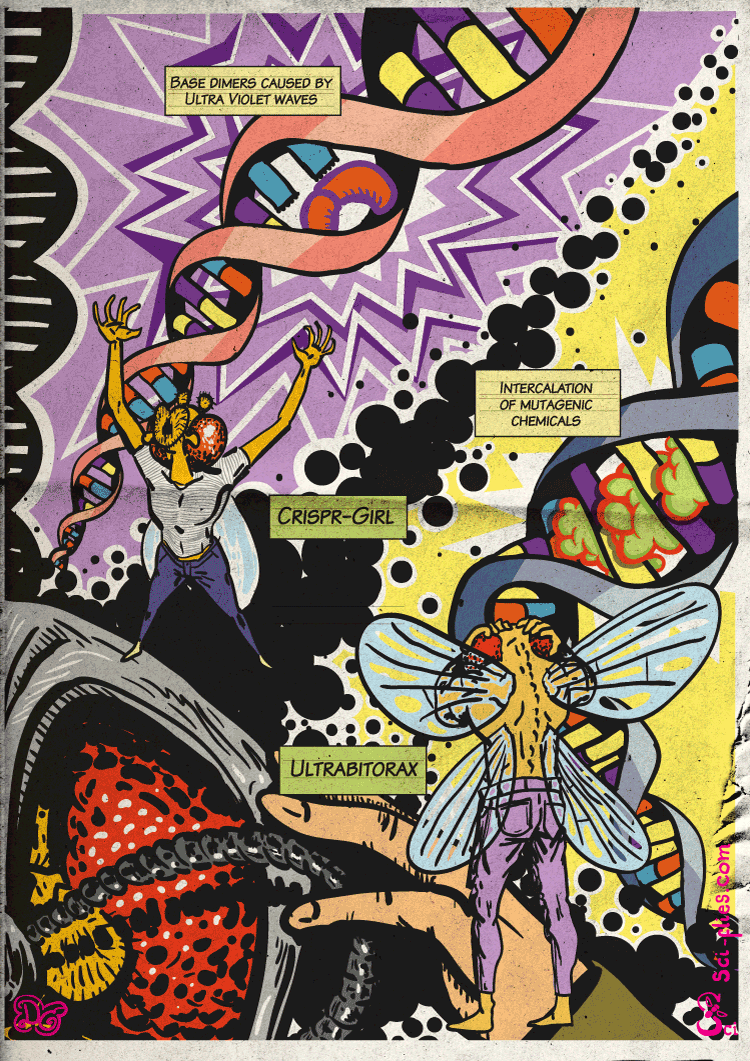
Consequences of mutations
So, what happens when mutations occur?
The effect of a mutation will depend on where in the genome the mutation occurs and on the nature of the mutation. In general mutations can have no effect, they can alter the product of a gene if they occur in coding sequences or they can alter the expression of the gene (where and when the product of the gene is made).
As we mentioned in the article about DNA structure and function, there is a genetic code to translate the information in DNA and go from a sequence of 4 elements (the bases) to proteins, that are composed of 20 different elements (amino acids). The code is composed of combinations of 3 bases that “mean” an amino acid. Since 4 elements can be combined in threes in 64 different ways (4x4x4), each amino acid is coded through more than one codon. The code is said to be redundant.
The redundancy of the code means that base substitution mutations may not change the protein at all. Also, even if the mutation produces a codon that changes an amino acid, many amino acids have similar properties and exchanging them will not affect the function of the protein in great measure.
Mutations that add bases or eliminate bases can have more drastic effects. Since the code is written in a single line of codons made of three bases each, eliminating or adding bases in numbers that are not divisible by three will provoke a complete change in the meaning of the sequence from the mutation onward. For example, if in the code TAG CTT ACT CGC… we eliminate the first A (only one base) we get TGC TTA CTC GC… completely different codons and a different meaning.
In any case mutations can be negative, neutral or positive, but most mutations that change proteins are harmful. Only a very small portion of the genomes of organisms such as flies and humans contain the code to make proteins (around 1.5% in humans), the rest has regulatory instructions to tell when and where proteins should be produced or codes to produce functional RNAs that do not make proteins or does nothing at all (around 20% in humans has no described function).
Regarding the visible effects of a mutation, we have to distinguish between genotype and phenotype. The genotype is the actual genetic information of an organism (the actual sequence of the DNA). The phenotype are the characteristics that can be observed. Many characteristics can constitute a phenotype. They can be physical, behavioral, molecular, anatomical, etc. In Drosophila flies, genes are usually named after the phenotypical characteristics observed when they are mutated.
For example, the white-eyed mutant fly Morgan found had a broken gene (genotype) that caused the eyes to be white (phenotype). The gene was named white. The phenotype at the physical level was white eyes, at molecular level the phenotype of that mutation is the absence of a protein that allows the molecules needed to produce red pigment to enter the cells of the eye (then the red pigment is not produced and the eyes are white).
Mutations can have many phenotypic effects at different levels. They can break a gene or make it work differently, enhancing its function or changing it.
To avoid harmful effects the organism has in place cellular and molecular mechanisms to check and correct errors in DNA replication and in its structure (we will talk about them at some other time). But as with replication itself, the mechanisms are not perfect and sometimes make mistake.
In summary we could say that the probability for a mutation to occur in important parts of the genome depends on the probability of the replication machinery to commit errors spontaneously, the probability of repair mechanisms to make mistakes and the amount of DNA in which mistakes are important. Generally, the mutation frequency is very low and there are environmental factors that can increase the frequency of errors significantly,
This is important because, from all of these factors, the ones we can better control are the environmental known mutagens such as the products of tobacco combustion or the excess of UV radiation. In somatic cells, mutations can provoke cancer when they occur in genes or in regions of the genome that control the processes of division and specification of cells. So, unless you have a mutant healing factor like Wolverine or Deadpool (which is highly unlikely) it is better to avoid smoking, and to use sunscreen.
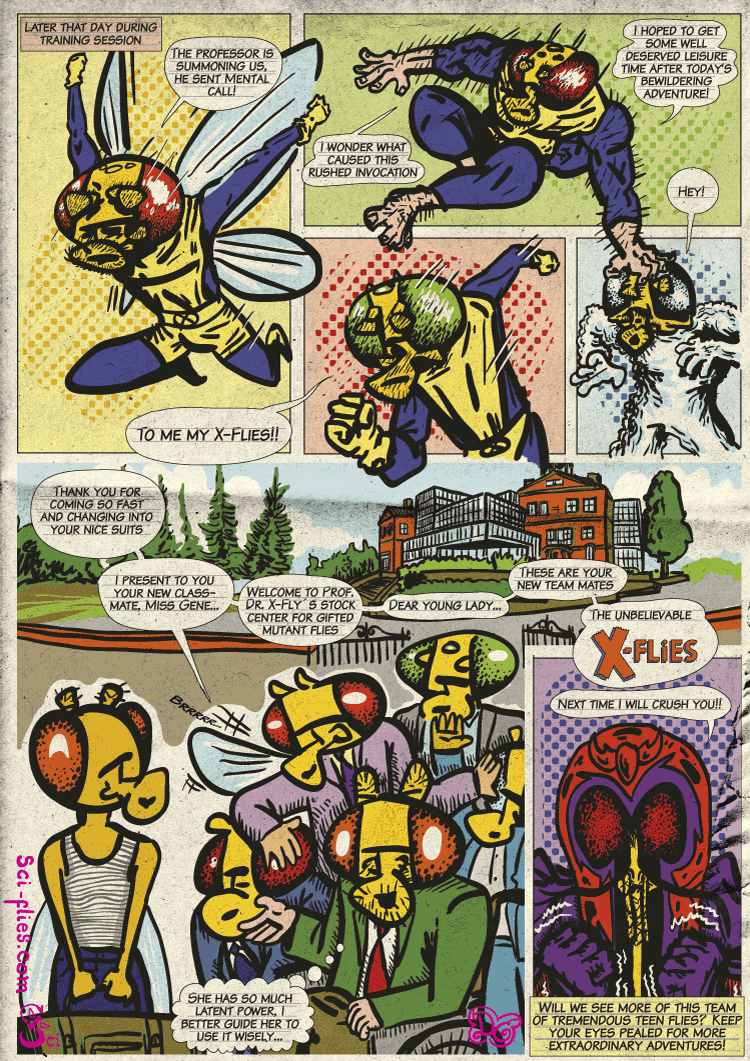
The Importance of mutations
After the millions of years of life on earth, why are mutations still happening? Why are these mechanisms making mistakes?
Mutations that can be passed down to a next generation of organisms (in the germ cells) are important in nature because they are the source of genetic variation. Through the many mechanisms described here come new variants. New genes or changes in how genes are used appear by mutation of existing DNA.
Since the environment in which organisms live is not constant in time (continents move, volcanoes erupt, climate changes, other organisms modify the environment or compete, etc) there is a need for novelties, variants that may be useful to overcome these uncertainties. Although many new variants may be harmful or even lethal, the positive effect of adding new useful variants to a population outweighs those loses in the long run. It is one of the most amazing and beautiful things about life, that all the diversity we know comes from randomness interacting with uncertainty.
As we have talked in many of the previous posts, mutations are important for scientific research. Not only to study them and the mechanisms of genetics and DNA function, but also to understand biological processes at other levels. As we saw when we talked about neurogenetics, damaging or modifying a gene or a regulatory region can reveal details about the biological processes, and not only genes.
For this reason, many techniques to make mutations have been developed. We have talked about some of them; the generation of random mutations and the engineering and insertion for DNA in the genome. Most recently a technique called CRISPR has started to allow targeting specific genes easily and fast, and to modify them more or less at will. This has caused a revolution beyond the research world due to its possible therapeutic applications and its ethical implications. On the next post we will explain how this technique works and what it can do.
See you next time!
Interesting References and comments
For this post I used as a source of information a very nice textbook, Genetics – A Conceptual Approach (5th Edition, 2014, by BJ Pierce).
You can read about Charlotte Auerbach’s life and career in the obituary published in Kilbey BJ, (1995) “Charlotte Auerbach (1899-1994)” Genetics 141(1): pp1–5. And you can read her original paper in Auerbach C, Robson JM & Carr JG (1947) Science 105(2723), pp243-247.
In this post I chose to reference my favorite superheroe comic books, the X-Men. I first knew them from the animated series in the 90s and started reading the comic books at around the same time. I consider this post a humble homage to their creator, Stan Lee, who recently passed away. He also created other characters, such as Spider-Man (another favorite of mine), the Hulk, Daredevil and the Fantastic Four.
An interesting aspect of his superheroe comic books in the context of this blog is that they were created in the early 60s, around the time of the molecular revolution in Biology. His heroes are all very rooted on the anxieties and amazement of the times regarding these discoveries (and also discoveries and developments in physics). The X-Men were mutants, Spider-Man was bitten by a radioactive spider, the Hulk had been bathed in gamma rays, Daredevil had an accident with chemicals and the Fantastic Four had been altered by radiation from cosmic rays in space. I find fascinating how these characters reflect a period of such fundamental discoveries.