CRISPR/CAS - On the Leading Edge
In the last post we defined what mutants are and how mutations occur. We have mentioned several ways in which we can generate mutations randomly and how we use them to investigate the genetic bases of biological processes (such as behaviors).
What happens then if we are interested in understanding a specific gene or a specific function of a gene? How can we break it or modify it directly to study its function? How can we target it without having to rely on random mutations?
In this post we will explore the latest techniques used to produce designed and targeted mutations. We will start with a conceptual explanation of the cellular machinery and biological mechanisms of which we take advantage to do this. Then we will go into the details of the CRISPR genome editing technique, which allows more precise and targeted mutations and modifications of the genome.
In the last post, I made up several characters referencing mutant flies (riffing on the Marvel Comics mutants, the X-Men). Two of them were not actually references to existing mutant flies but were references to techniques for transgenesis or genome modification.
The character “Eyeless” (the stand-in for the X-Men’s Cyclops) was a transgenic fly expressing GFP in the eyes, as we learned in the posts about transgenic flies and enhancer traps. The second one, CRISPR Girl (a stand-in for the x-men’s own Jean Grey AKA Marvel Girl and later on in its character history Phoenix) refers to the CRISPR genome editing technique. As we explain this technique, we will get to know CRISPR girl’s powers.
As you know, with great power comes great responsibility.
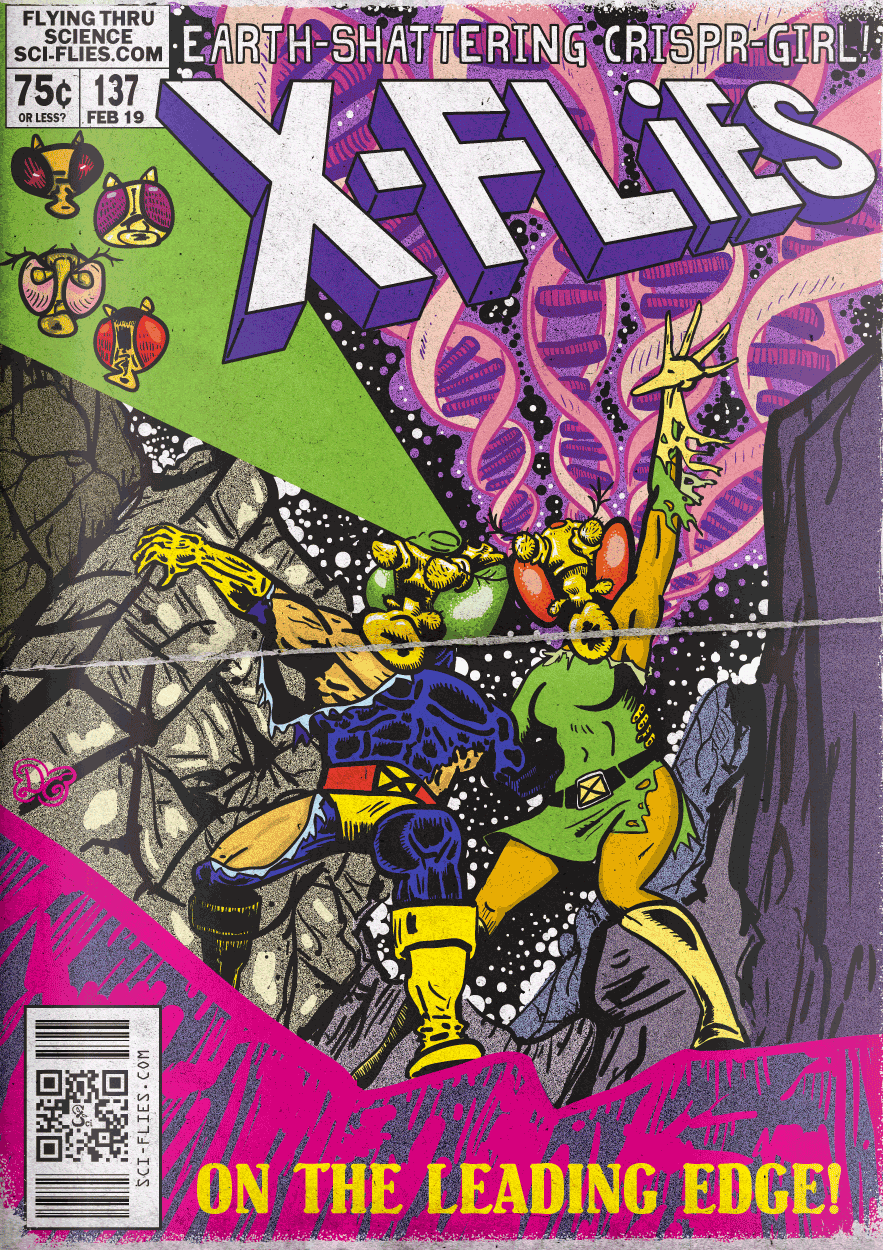
The Mechanisms for DNA Repair
The DNA molecule carries information in its sequence to determine how and when to build the many different RNAs and Proteins that perform all the functions of a cell. For this reason, it is very important to maintain this information unmodified.
However, it happens to be that the DNA molecule is under constant attack by damaging factors. On one hand, the same regular function of the cells generates molecular byproducts that can damage DNA. In addition, organisms are constantly exposed to external agents such as radiations and chemicals that can have DNA damaging activity. Damage to DNA can result in mutation of its sequence and posterior alteration of cellular functions.
Not to worry though, to minimize the effects of DNA damage the cell has mechanisms that check and repair the integrity, structure, and sequence of the DNA molecule.
One of the most dramatic types of damages to the DNA molecule is the double strand brake. This implies the complete break of the molecule in two, leaving free ends. Double strand breaks can result in the death of a cell if they go unrepaired.
To deal with double strand breaks the cell has two basic types of mechanisms.
The first type of repair mechanism is called Non-Homologous End Joining (NHEJ), and it does just that, it joins the ends of the broken strands and fuses them again as they are (here’s a video). This mechanism is very error prone, because when DNA breaks, parts of the sequence may be lost or rearranged (from one to several bases) and the repair may result in deletions or insertions with consequent possible loss of gene function (as we described in the post about mutations).
The second type of mechanism is called Homology Directed Repair (HDR). The basic concept of HDR is that it uses an available, second, unbroken copy of the DNA molecule (called a homologue sequence) as a template to copy and repair the broken sequence, thus avoiding errors and missing parts (here’s a video). Cells normally have a second identical copy of their DNA during the period of their life when they are replicating it or when they have just finished replicating it (we will talk about the life of the cell, the cell cycle, some day).
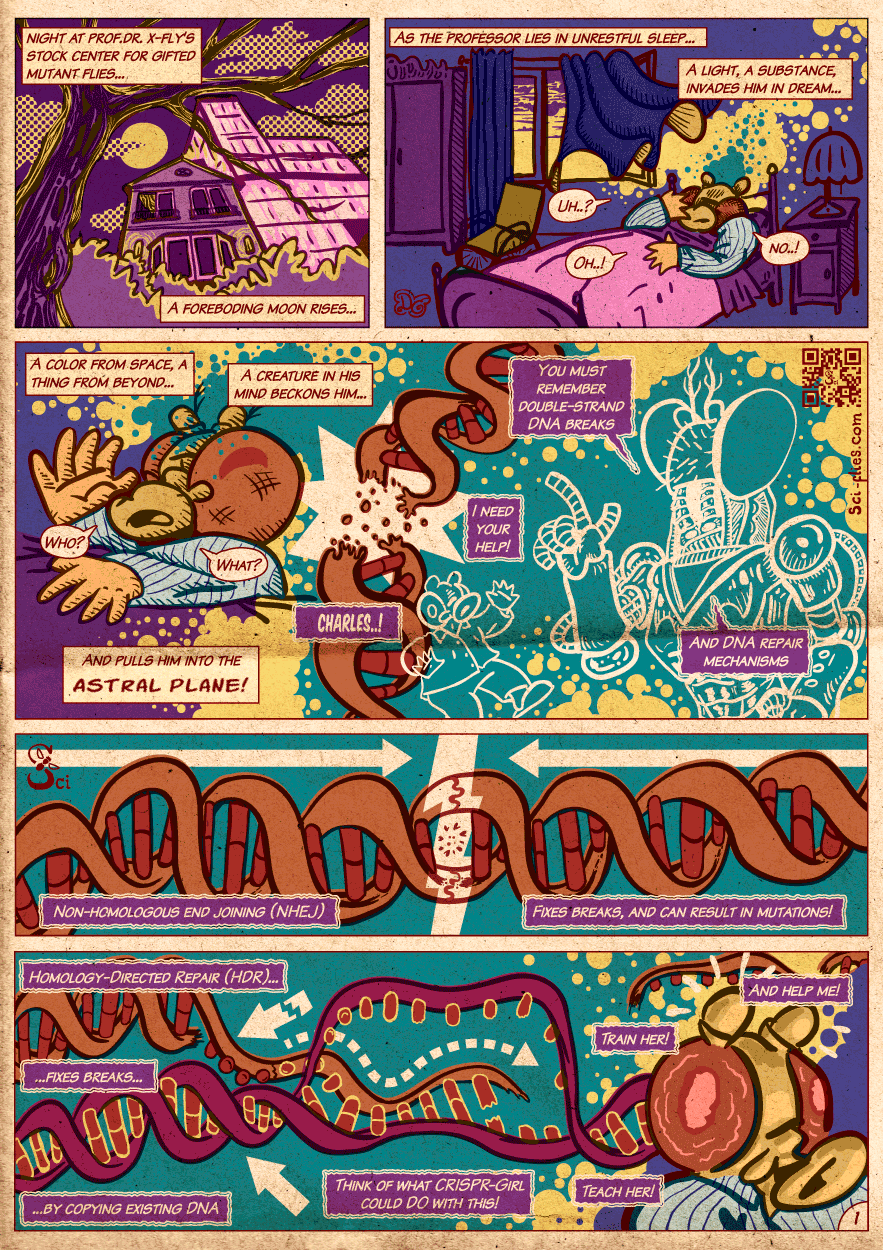
How can we take advantage of the DNA repair mechanisms to generate mutations?
For once we could induce a double strand break on the gene of interest and the NHEJ repair may cause mutations in it such as insertions or deletions, hopefully knocking it down (making it not functional).
Or we could use the HDR machinery to make custom modifications to the DNA sequence of the cell, that is, to edit the genome. Since HDR repairs the broken sequence by copying the sequence of a template into the break, we could make a custom DNA in the lab that contains the characteristics that allow the cell to use it as a template but put in it some sequences we would like to insert. In this way we could trick the HDR mechanism into introducing edits into the genome (what types of edits you ask, just keep reading).
But how do we make directed double strand breaks? Until now we have only talked about ways of doing it randomly with X-Rays and Chemicals.
How do we break the DNA at the gene we want to knock down or at the place where we want to make an edit?
Targeted Double Strand-Breaks
Directing modifications to specific sites of the genome has long been a dream and a complicated task to achieve. There are two main requirements that a mechanism or technique for the generation of targeted breaks in DNA has to fulfill:
- It needs a customizable targeting system. A way to be able to recognize only the specific sequence of the DNA we want to cut, amongst all the rest of the DNA in the genome (and also not get confused with other similar sequences that may exist).
- It needs to be able to cut the DNA in the target sequence or in its vicinity, so that we know exactly where the break occurs.
In cells, the proteins are the molecules that do things, from giving structure to performing chemical reactions. Throughout the history of cellular and molecular biology many mechanisms in cells have been described involving proteins that can interact with or modify DNA and RNA and we have learned to use them as tools in the lab.
The most recent approaches to our issue used engineered proteins made up of a fusion of proteins that carry out these two tasks independently:
- DNA binding proteins that can recognize and bind specific DNA sequences.
- Nucleases that can cut DNA
These fusion proteins can target a sequence thanks to the DNA binding activity and cut it thanks to the nuclease (the two existing techniques are the ZFN – Zinc Finger Nucleases and the TALEN – Transcription Activator-Like Effector Nucleases). The problem with these approaches is that it is a hard task to design and produce the proteins that can bind to specific sequences of DNA.
A normal function of some of these DNA binding proteins (the Zinc Finger or the Transcription Activator-Like Effectors) is to recognize short, specific sequences in the genome located in the vicinity of the genes, bind to them and activate the machinery that regulates gene expression. We have learned to use them and engineer them to make them recognize any custom sequence.
Another technique, called Gene Targeting, was the first one used to generate mutants of specific genes. This technique also uses the HDR machinery to introduce modifications in the genome from a donor DNA, but it does so without provoking cuts in the target DNA, greatly reducing its efficiency.
The development of the Gene Targeting technique in mice (during the early 80s) was the subject of the 2007 Nobel Prize awarded to Capecchi, Evans and Smithies. The technique was first put to use in flies in the early 2000s.
The CRISPR/Cas system
The complications of the previous techniques were solved by the use of a different system called CRISPR/Cas.
In nature, the CRISPR/Cas system functions in certain types of bacteria as a sort of acquired immunity mechanism. These bacteria are infected by viruses that inject their DNA into them. The viral DNA hijacks the bacterial system to make more viruses. To defend itself, the bacteria need to be able to recognize these specific viral DNAs, target them and destroy them. In a way, the bacteria face the same problem as the molecular biologist, targeting a specific sequence and not a random one to break it.
Millions of years of evolution, battling viruses, have given the bacteria a solution to the problem. When foreign DNA enters the bacteria, it is chopped down and pieces are incorporated in a region of the bacterial genome called the CRISPR locus. These DNA pieces are then used to make small RNAs that have the same sequence as the viral DNA (they are complementary to the viral DNA), these are called crRNAs. The CRISPR locus also has a gene to make a protein called Cas (CRISPR Associated), which has nuclease activity (cuts DNA) and an adaptor RNA called tracrRNA.
Using the adaptor tracrRNA the Cas protein can grab the different small crRNAs. These small RNAs will serve to guide the Cas protein to the viral DNAs that match the sequence of the guides. Then through its nuclease activity, the Cas protein cuts the viral DNA and inactivates it.
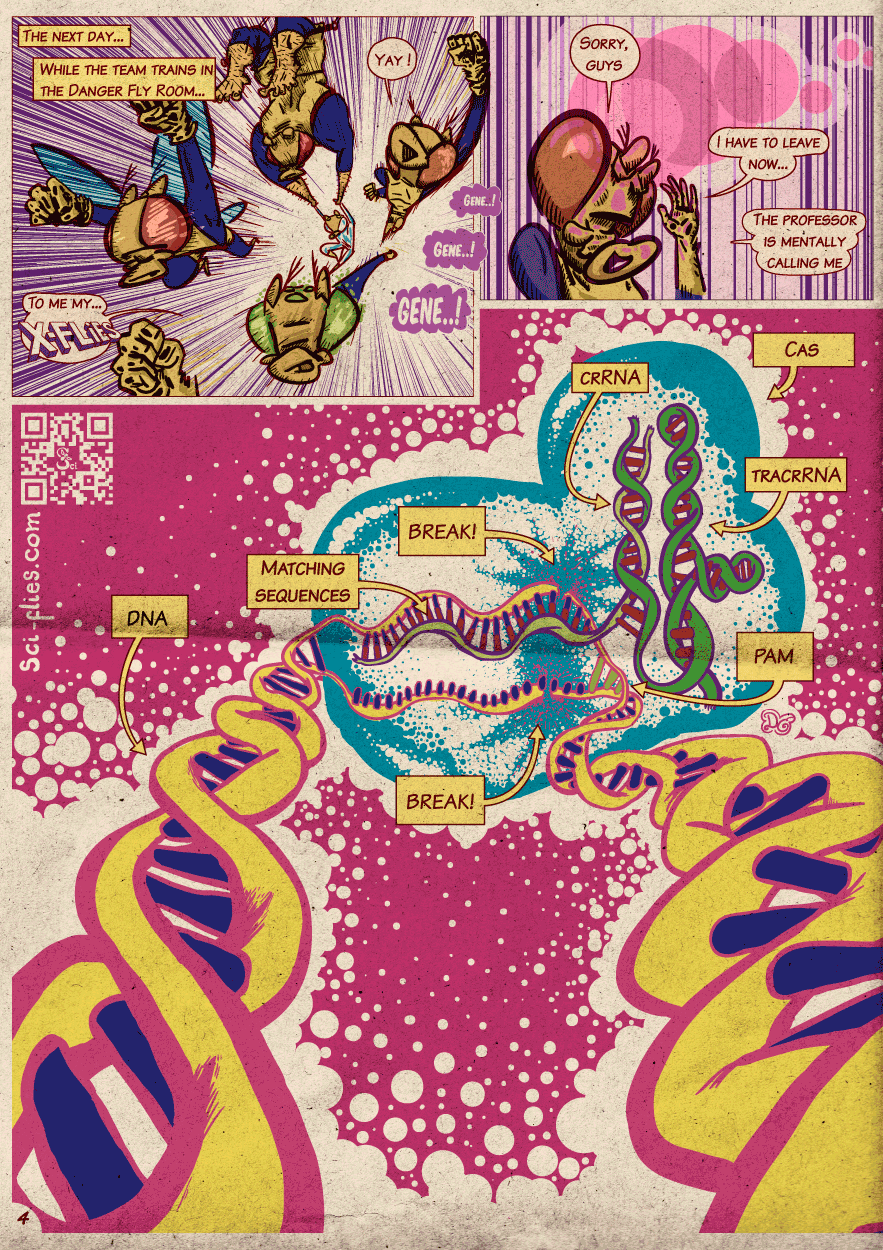
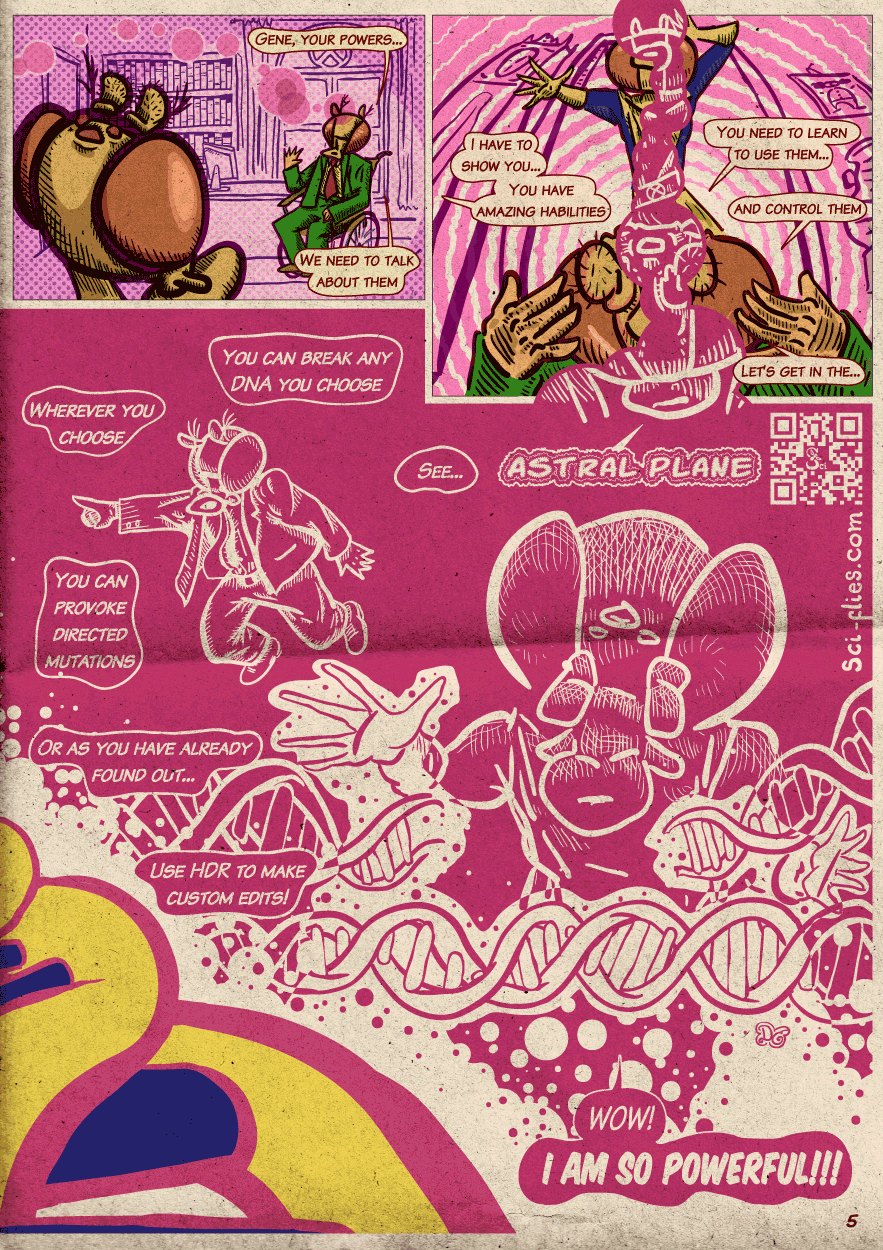
The use of CRISPR/Cas in genetic engineering
Summing up what we have learned until now, the best way to make targeted mutations or edition of genomes is to produce a targeted double strand break. Then, either we hope for mistakes by the NHEJ machinery of the cell or we provide it with an engineered template that contains the edits we want to introduce to sneak them in through the HDR repair process.
The CRISPR/Cas system has all we need to easily and accurately make site-directed double strand breaks: the DNA cutting machine and targeting mechanism. And this is done simpler than with the protein-based methods used before.
The beauty of the CRISPR/Cas system lies in the targeting mechanism. It is based on RNA sequences instead of proteins. The RNA guides match the DNA sequence they target one-to-one, so designing them is very straight forward and easy. They can be produced in any lab and require only common molecular biology reagents and techniques.
There is a pleasing rhyme in the way the CRISPR/Cas system works. All three main components of the sequence-based genetic machinery combine to walk a backwards trail and allow the modification of the sequence that keeps the genetic information in the first place.
As we know DNA holds the information to code for RNA to make proteins that have biological activity. In the CRISPR/Cas technique, the cutting activity of a protein is guided by an informative RNA to the DNA database to modify it.
The use of the technique follows these steps:
- Define the sequence to target.
It cannot be any sequence. The protein has a few restraining requirements for sequence recognition, but these are not limiting and there are many programs, apps and on-line tools that search for these sites in a given DNA sequence.
The most important constraint is that there has to be a specific three “letter” (nucleotides) sequence preceding the guide/target (called a PAM sequence), but the characteristics of this sequence make it very frequent in the genome.
- Design and produce guide RNAs (crRNAs and tracrRNAs or a combination of both called gRNAs).
The most important aspect of designing a guide is that it has to bind only to the site we want to target. The Cas protein must cut where we want and only where we want so there should not be any sequence in the whole genome that is similar enough to be mistaken with our target (sequences similar to the target are called off-targets).
To aid on the search for the perfect target site, there are many software tools that check and compare the possible target sequences with the sequence of the whole genome we are targeting (Drosophila, human, mouse, etc).
- Optionally, produce a DNA template for HDR repair and genome editing.
In the lab we can make copies of DNAs from different sources, cut them and paste them together. In this way, we can make modified versions of a gene of interest to alter its product (protein or RNA). We can generate versions that produce inactive or less active proteins. Or versions with an enhanced activity. Or with an altered localization within the cell. We can make genes that produce fluorescent versions of their proteins. And we could also modify the regions of the genome that regulate gene expression, to alter when and where a gene is expressed. And many more modifications.
The strategy to edit the genome through HDR consists in sneaking modifications within the DNA that can be used by this repair machinery to stitch the doubly broken strand back together. As we mentioned above, this machinery looks for DNA that is the same as the broken one, to copy and insert it.
What we do is place on both sides of the DNA we want to introduce, sequences that match the ones around the break point. The machinery will then pair the frayed ends of the break to the matching sequences of both sides of our edited DNA. Then it will fill in the gap between the ends of the break with the DNA we provided, because it assumes that the DNA we provided is the undamaged version of the damaged DNA.
- Introduce this in the target organism together with a source of Cas protein.
This is a very critical point that can make the technique harder to use in some species than in others. For each different species of organism, the way to introduce the molecular tools for transgenesis or gene editing (DNA or Proteins) will vary.
We have mentioned before that to introduce engineered DNA in the genome of an organism and make it heritable, we have to do it in cells that can later on produce a whole organism.
In flies (as we discussed in the post about transgenics) we introduce foreign DNA in the genome by injection in fly embryos, where it can get inside the cells that will later produce sperm and eggs (the germ cells).
All these steps have variants, modifications or additional steps to make them more efficient for specific objectives, but the general workflow goes along these lines.
The use of the bacterial CRISPR/Cas system as a technique for making edits and mutations in other organisms was first published in 2012-2013. In those years the technique was used in many model organisms; isolated human cells, roundworms, Drosophila flies, zebra fish, etc.
Since then, new tools are in constant development to make the CRISPR/Cas system more efficient and easier to use; The Cas protein has been modified to make it better, the software tools to find good target sequences are faster and more precise. Even many private companies now specialize on the technique and offer researchers the services to make all or part of the work for a fee.
Power and responsibility
For scientific research, the CRISPR/Cas technique is a dream-come-true. It allows us to easily make detailed modifications to specific genes and test their function in living organisms. We can now transfer and test the knowledge we have gathered about genes and their functions in model organisms (such as our beloved Drosophila melanogaster flies) to other less studied organisms, in a direct and targeted manner. This is a great tool to produce more and better basic knowledge on how living organisms work and evolve.
Using as an example a gene we have talked about before, if we know the sequence of the Period gene in other insect species, we could make targeted mutants and test if this gene has a similar function in those species as in Drosophila flies. The possibilities are endless.
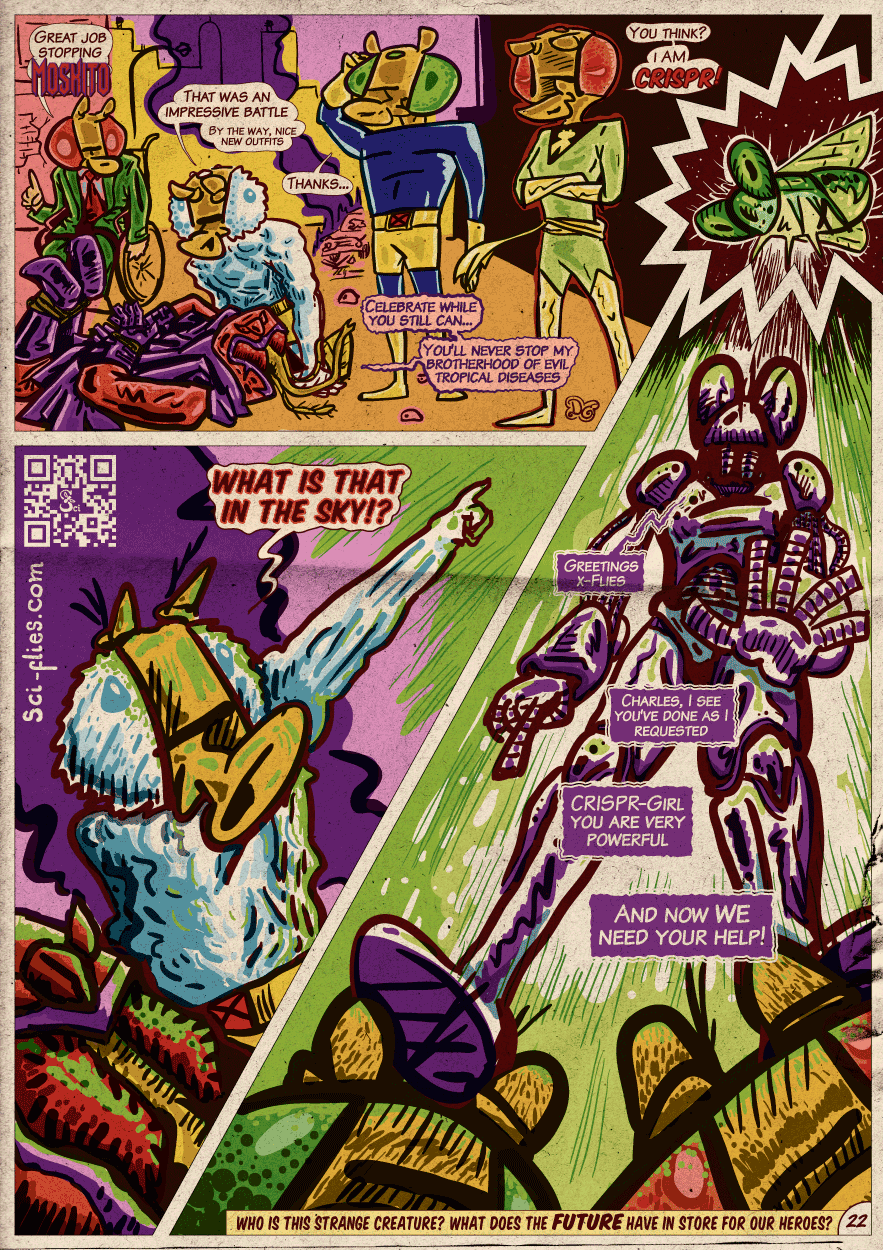
But what about our daily life? Can this technology have practical applications to improve our quality of life?
The CRISPR/Cas technique turns genome editing into a very accessible task. This could have a great impact in applications such as food production and human health. In fact, this potential has already sparked a long legal battle for the patent and the control over its exploitation.
In agriculture, this technology could serve to improve crops and livestock both for increased production and resistance to adverse conditions or for pest control. In medicine to target gene variants responsible for genetic disorders, for fixing mutations in cancer through gene therapy or for controlling animals (such as insects) that transmit diseases. And it does not have to stop there.
As with any technological development, the application of this technique will require to balance benefits and risks. We need to discuss a universal ethical framework to define those benefits and risks (health, economical, societal, environmental, etc) and weight them to strike that balance. I will leave you with some questions in this regard. I encourage you to think further questions, risks and benefits.
Who will have access to the use of this technique, to its products and its benefits?
How will the modified organisms impact the environment in which they are introduced?
How will modified crops and animals affect small producers and regional economies?
What will modified organisms be used for and who will monitor their use?
Should we make heritable modifications in humans? If so, in which cases?
The CRISPR/Cas technique is more efficient, precise, cheap and easier to apply than previous techniques. The need to discuss the regulation of its use is urgent. Going no further, very recently a scientist in China claimed to have made the first heritable modifications in humans. In embryos, he mutated the human gene that produces a protein needed for the HIV virus to infect. The human babies born are then potentially immune to HIV, modified through a new technique that is still being refined and tested.
What we have to keep in mind is that this technology is here to stay. It will continue to be developed and will be swiftly put to use. It is then important for international collaboration to set up the appropriate regulations so that we use it in a way that guarantees respect for all human rights and environmental sustainability.
To continue in the comic book vein, the CRISPR/Cas technique gives us a great power that requires great responsibility.
Next post we will go back to biology and talk about development and what we can learn from the fly embryo.
References, comments and Traiding Cards!
Scroll to the end for a bonus!
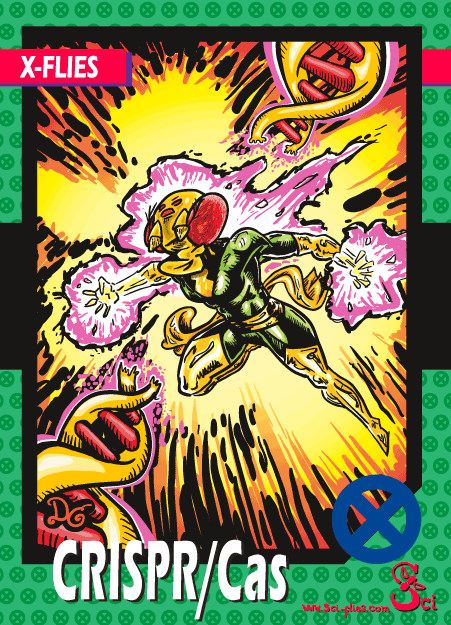
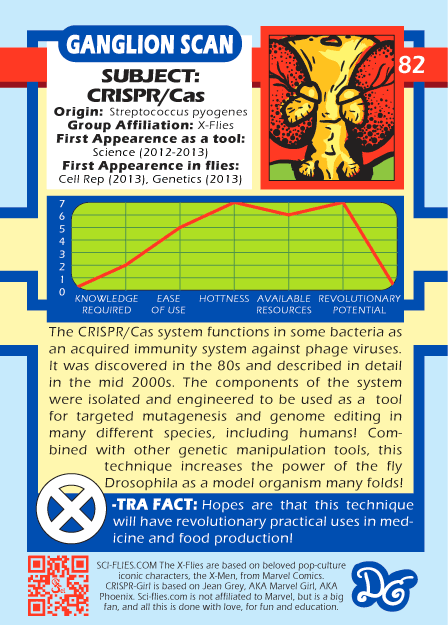
This post is the sibling of the previous one (you will have noticed that my posts come in pairs), in both I chose to make reference to my favorite superhero comics, the X-Men. In this second part, I mix a bit the original 60s X-Men with the revamped X-Men from the 70s-80s. In 1970 the series was canceled, only to return later, with Chris Claremont as scriptwriter, in 1975. New characters were introduced (now iconic, such as Storm, Wolverine, Colossus and Nightcrawler) and the stories became more dramatic, with trips to space and time travel. Jean Gray became Phoenix, an interstellar being of great power, which then becomes corrupted and turning into a villain, the Dark Phoenix …
For this post I used as a source of information several reviews and papers. I have brushed the many subjects on the surface, so I recommend this as a starting point if you want to read more in depth (also the Wikipedia links in the text).
CRISPR in genetic engineering
A Programmable Dual-RNA-Guided DNA Endonuclease in Adaptive Bacterial Immunity – Jinek, et al. Science, Vol. 337, Issue 6096, pp. 816-821 (2012) https://doi.org/10.1126/science.1225829
Multiplex Genome Engineering Using CRISPR/Cas Systems – Le Cong, et al. Science Vol. 339, Issue 6121, pp. 819-823 (2013) https://doi.org/10.1126/science.1231143
RNA-programmed genome editing in human cells – Jinek et al. eLife 2:e00471 (2013) https://doi.org/10.7554/eLife.00471
RNA-Guided Human Genome Engineering via Cas9 – Mali et al. Science, Vol. 339, Issue 6121, pp. 823-826 https://doi.org/10.1126/science.1232033
On the fight over patenting
How the battle lines over CRISPR were drawn – Un muy buen resumen sobre la historia del desarrollo de la técnica y las facciones involucradas en batalla legal por patentarla.
https://www.sciencemag.org/news/2017/02/how-battle-lines-over-crispr-were-drawn
In Drosophila
Genome engineering of Drosophila with the CRISPR RNA-guided Cas9 nuclease – Gratz el al. Genetics Vol. 194, pp. 1029–1035 (2013) https://doi.org/10.1534/genetics.113.152710
Highly Efficient Targeted Mutagenesis of Drosophila with the CRISPR-Cas9 System – Basset et al. Cell Reports, Vol. 4, pp. 220–228 (2013) http://dx.doi.org/10.1016/j.celrep.2013.06.020
The mutagenic chain reaction: A method for converting heterozygous to homozygous mutations – Gantz & Bier. Science, Vol. 348, Issue 6233, pp. 442-4. (2015) http://doi.org/10.1126/science.aaa5945
Gene targeting
Gene targeting by homologous recombination in Drosophila – Rong & Golic. Science. Vol. 288, Issue 5473, pp.2013-8 (2000). https://doi.org/10.1126/science.288.5473.2013
Targeted mutagenesis by homologous recombination in D. melanogaster – Rong, et al. Genes & Development. Vol. 16, Issue 12 pp.1568-81 (2002) https://doi.org/10.1101/gad.986602
Reviews about Gene Targeting, ZNF, TALEN y CRISPR
ZFN, TALEN, and CRISPR/Cas-based methods for genome engineering – Gaj, et al. Trends in Biotechnology. Vol. 31, Issue 7, pp. 397-405 (2013) https://doi.org/10.1016/j.tibtech.2013.04.004
Strategies for gene disruption in Drosophila – Lin, et al. Cell & Bioscience Vol. 4, Issue 63, (2014) https://doi.org/10.1186/2045-3701-4-63
Reviews about CRISPR in bacteria
CRISPR/Cas, the Immune System of Bacteria and Archaea – Horvath & Barrangou. Science Vol. 327, Issue 5962, pp. 167-70. (2010) https://doi.org/10.1126/science.1179555
CRISPR interference RNA-directed adaptive immunity in bacteria and archaea – Marraffini & Sontheimer. Nature Reviews Genetics. Vol. 11, pp. 181-90 (2010) https://doi.org/10.1038/nrg2749
CRISPR-Cas System and Its Role in Phage-Bacteria Interactions – Deveau, et al. Annual Review of Microbiology. Vol. 64, pp. 475–93 (2010) https://doi.org/10.1146/annurev.micro.112408.134123
CRISPR in Genetic Engineering Reviews
Development and Applications of CRISPR-Cas9 for Genome Engineering – Hsu, et al. Cell, Vol. 157, Issue 6, pp. 1262-78. (2014) https://doi.org/10.1016/j.cell.2014.05.010
The new frontier of genome engineering with CRISPR-Cas9 – Doudna & Charpentier. Science, Vol. 346, Issue 6213, 1258096 (2014) https://doi.org/10.1126/science.1258096
CRISPR-Cas systems for editing, regulating and targeting genomes – Sander & Joung. Nature Biotechnology, Vol. 32, pp. 347–355 (2014) https://doi.org/10.1038/nbt.2842
Molecular biology at the cutting edge: A review on CRISPR/CAS9 gene editing for undergraduates – Thurtle-Schmidt & Lo. Biochemistry and Molecular Biology Education, Vol. 46, Issue 2, pp. 195-205. (2018) https://doi.org/10.1002/bmb.21108
CRISPR/Cascade 9-Mediated Genome Editing-Challenges and Opportunities – Roy, et al. Frontiers in Genetics. Vol. 9, 240 (2018), https://doi.org/10.3389/fgene.2018.00240
Ethic and legal issues
Ethical Issues in Genome Editing using Crispr/Cas9 System – Rodriguez. Journal of Clinical Research & Bioethics, Vol. 7, Issue 2 (2016) https://doi.org/10.4172/2155-9627.1000266
CRISPR Ethics: Moral Considerations for Applications of a Powerful Tool – Brokowski & Adli. Journal of Molecular Biology, Vol. 431, Issue 1, pp. 88-101 (2018) https://doi.org/10.1016/j.jmb.2018.05.044
Gene drives in our future: challenges of and opportunities for using a self-sustaining technology in pest and vector management – Collins. BMC Proceedings. Vol. 12, Suppl. 8 (2018) https://doi.org/10.1186/s12919-018-0110-4
Genome editing of crops: A renewed opportunity for food security – Georges & Ray. GM Crops & Food, Vol. 8, Issue 1 (2017) https://doi.org/10.1080/21645698.2016.1270489
Normative Criteria and Their Inclusion in a Regulatory Framework for New Plant Varieties Derived From Genome Editing – Hamburger. Frontiers in Bioengineering and Biotechnology. Vol. 6, 176. (2018) https://doi.org/10.3389/fbioe.2018.00176
News, opinions and interesting articles
National Geographic on CRISPR and Crops modification. Why Gene Editing Is the Next Food Revolution. https://www.nationalgeographic.com/environment/future-of-food/food-technology-gene-editing/
2015 – Don’t edit the human germ line https://www.nature.com/news/don-t-edit-the-human-germ-line-1.17111
2015 – Ethics of embryo editing divides scientists https://www.nature.com/news/ethics-of-embryo-editing-divides-scientists-1.17131#/b1
2016 – CRISPR gene-editing tested in a person for the first time https://www.nature.com/news/crispr-gene-editing-tested-in-a-person-for-the-first-time-1.20988
2018 – Chinese researcher claims to have created gene-edited twins. https://www.sciencemag.org/news/2018/11/crispr-bombshell-chinese-researcher-claims-have-created-gene-edited-twins
2018 – George Church, head of one of the labs that developed the CRISPR/Cas technique gives his opinion on gene edited babies https://www.sciencemag.org/news/2018/11/i-feel-obligation-be-balanced-noted-biologist-comes-defense-gene-editing-babies
2019 – A very, very interesting article on the events of the Second International Summit on Human Genome Editing (Washington DC, 2018), where He Jiankui, from China, announced that he had created the first CRISPR edited humans. I highly recommend reading it, if not for the engaging account of the event, for the extremely thoughtful evaluation of the matter. We need not fear this technology. As researchers and service providers we should be clear, open and serious in its handling. As beneficiaries we should understand it and use it responsibly http://doi.org/10.1242/dev.175778
2019 – News on the reaction of Chinese authorities on the news of these developments. He was fired https://www.nature.com/articles/d41586-019-00246-2
2019 – China tightened rules, regulations and penalties. https://www.nature.com/articles/d41586-019-00773-y
BONUS! the very, rare very though after, golden metallic variant of the CRISPR/cas trading card
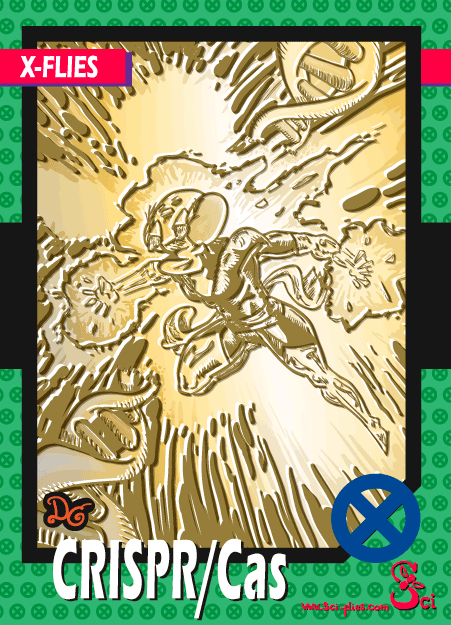
Hey, you got this far! Why not leave a comment below? Or on our Facebook or Instagram! Let me know if you liked the post or the drawings.